Perspectives
Hepatic erythrophagocytosis connects systemic metabolic deregulation to iron overload and inflammation
In this issue of Endocrinology and Metabolism, in the special collection of Immunometabolism, Chen et al discuss the role of iron metabolism and ferroptosis in nafld. The authors nicely describe the molecular mechanisms regulating the iron levels and the susceptibility to ferroptosis. However, their viewpoint reflects that of the scientific community on ferroptosis: they underestimate the role of hepatic erythrophagocytosis. In this commentary we are trying to elaborate on the role of hepatic erythrophagocytosis in the progression of NAFLD.
In healthy state, red blood cells are mainly phagocytosed by the spleen, and to some extent by the liver. However, under extended erythrocyte damage the liver undertakes the mission of eliminating red blood cells. During NAFLD metabolic changes in the circulation influence erythrocytes. Previous studies have shown that exposure of red blood cells in an environment with high levels of lysophosphatidylcoline, lysophosphatidic acid, sphingosine phosphate, ceramide, cholesterol and glucose impairs erythrocyte function. This can then lead to erythrophagocytosis. Indeed, Otogawa et al showed that in NASH animal models there is increased erythrocyte phosphatidylserine exposure. This lipid is recognized by the opsonin lactadherin, whose levels are increased by exposure of macrophages to high levels of fatty acids. Erythrocyte opsonization by lactadherin then drives hepatic erythrophagocytosis. This was followed by hepatic iron overload, and lipid peroxidation, a marker of ferroptosis. The authors of that study also showed that in livers of NAFLD patients there is increased erythrocyte accumulation. Similar results were recently reported by Park et al. They show that there is increased hepatic erythrophagocytosis in nafld animals. This was followed by macrophage and hepatocyte ferroptosis. Mechanistically, they showed that erythrocyte accumulation in the liver is preceded by platelet accumulation. Ultimately, interaction between erythrocytes and platelets was found to drive erythrocyte phosphatidylserine exposure and erythrophagocytosis. Attenuation of erythrophagocytosis inhibited ferroptosis. These studies, along with studies in model animals of atherosclerosis show that increased erythrophagocytosis augments the levels of delivered iron, rendering macrophages incapable of manipulating iron despite the increased expression of heme – oxygenase .
However, the detailed molecular mechanisms are far from elucidated. Nevertheless, our group has made efforts on elaborating on the signals that drive erythrophagocytosis in NAFLD. In particular, our unpublished data indicate that various opsonins including lactadherin, calreticulin, mannose binding lectin, MCP1, but not thrombospondin-1, are implicated in erythrophagocytosis during human NAFLD. In addition, we have also shown that erythrocytes of NAFLD patients contain decreased levels of CD47, the major signal of self in erythrocytes. Collectively, increased hepatic erythrophagocytosis contributes to hepatic iron overload in NAFLD, and various mechanisms can drive this process.
Although increased hepatic erythrophagocytosis per se can lead to hepatic iron overload and ferroptosis, there are studies indicating that iron alone is not sufficient to trigger ferroptosis. In particular, a recent study reported that phagocytosis of erythrocytes containing high levels of lipid peroxides but not of healthy erythrocytes drives ferroptosis. Indeed, several findings support that the environment of the phagocyte influences its susceptibility to iron overload and ferroptosis. Interestingly, a recent study showed that erythrocyte-derived IL-33 has the ability to trigger the development of iron-recycling macrophages. Importantly, lack of erythrocyte -derived IL-33 led to iron overload in the spleen and liver. Erythrophagocytosis -depenedent iron overload could also be affected by the erythrocyte content of ferroportin; Lack of erythrocyte ferroportin leads to hepatic iron overload.
In addition, there are reports showing that erythrophagocytes are anti-inflammatory. This could doubt the previous studies. Relatively, Pfeferle et al, recently showed that antibody – dependent hepatic erythrophagocytosis triggers the development of anti-inflammatory erythrophagocytes in the liver. Importantly, this protected model animals from developing NAFLD. However, this could be explained by the mode of erythrocyte death; Heme signaling inside the phagocyte can activate NRF-2 , but on the cell membrane could activate TLR4. In addition, on a cellular level, antibody-mediated erythrophagocytosis leads to a more rapid erythrolysosome formation.
Considering that erythrophagocytosis during NAFLD refers to intact erythrocyte phagocytosis, what renders this step pro-inflammatory? Although the metabolic cargo of red blood cells could certainly play a significant role, we should also keep in mind that there was recently discovered another erythrophagocyte that is pro-inflammatory. In particular, it was found that repeated stimulation of TLR9 or TLR7 can drive the development of a specific macrophage subset that is pro-inflammatory and performs phagocytosis of damaged erythrocytes. Accordingly, we recently reported augmented TLR9 levels on erythrocytes of NAFLD patients. These TLR9 molecules could deliver mitochondrial DNA on hepatic macrophages and drive their differentiation to pro-inflammatory erythrophagocytes.
Overall, hepatic erythrophagocytosis can be either anti- of pro- inflammatory, depending on the the mode of death, the levels of IL-33 and TLR9.
To sum up, erythrocytes can deliver an augmented iron cargo to hepatic macrophages in NAFLD, along with signals rendering these cells pro-inflammatory. We propose here that the extent and molecular mechanisms of hepatic erythrophagocytosis in NAFLD should be taken into consideration when examining hepatic iron overload and ferroptosis.
Immunometabolic role of intratumor erythrocytes
Dr. Charalampos Papadopoulos has proposed that intratumor erythrocytes are exposed in a milieu of low glucose and glutamine, and high lactate and lysophosphatidic acid concentration. He hypothesizes that reduced availability of glutamine and glucose would result in impaired antioxidant capacity, while lactate would further diminish ATP synhtesis. Lysophosphatidic acid could lead to erythrocyte programmed death. ROS along with erythrophagocytosis in this context could amplify immunosuppresion in the tumor microenvirinment.
Papadopoulos, C. Immunosuppressive Function of Intratumor Red Blood Cells: An Immune-Metabolic Perspective. Curr. Cancer Rev. 2022, 18, 224–226.
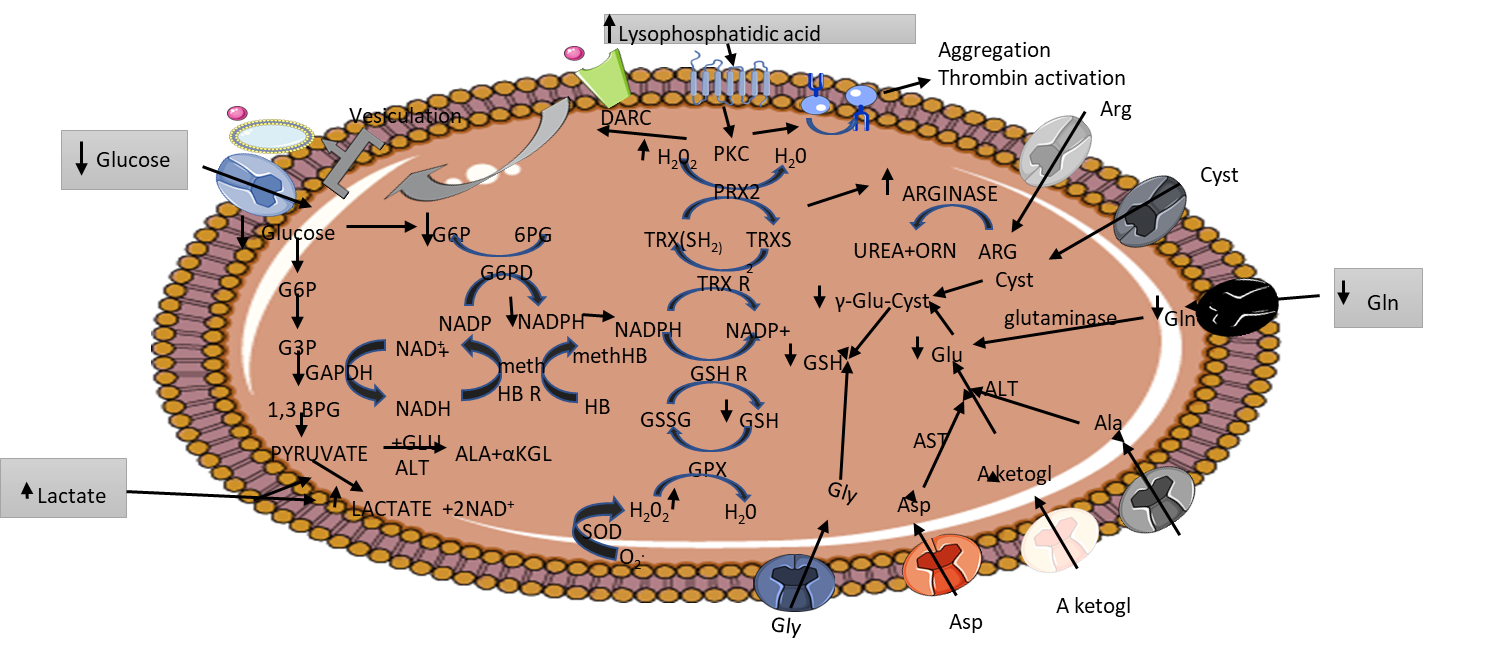
Systemic lipotoxicity and glucotoxicity lead to vascular inflammation via erythrocytes
Dr Charalampos Papadopoulos has gathered all the evidence which show that accumulation of both toxic lipids and sugars result in vascular inflammation and dysfunction. Arginase activity, erythrophagocytosis, thrombin generation and abrogated deformability are the main mediators for the lipotoxicity- and glucotoxicity-induced vascular inflammation.
Papadopoulos, C.; Tentes, I.; Anagnostopoulos, K. Lipotoxicity Disrupts Erythrocyte Function: A Perspective. Cardiovasc. Hematol. Disord. Drug Targets 2021, 21, 91–94.
Papadopoulos, C. Erythrocyte Glucotoxicity Results in Vascular Inflammation. Endocr. Metab. Immune Disord. Drug Targets 2022, 22, 901–903.
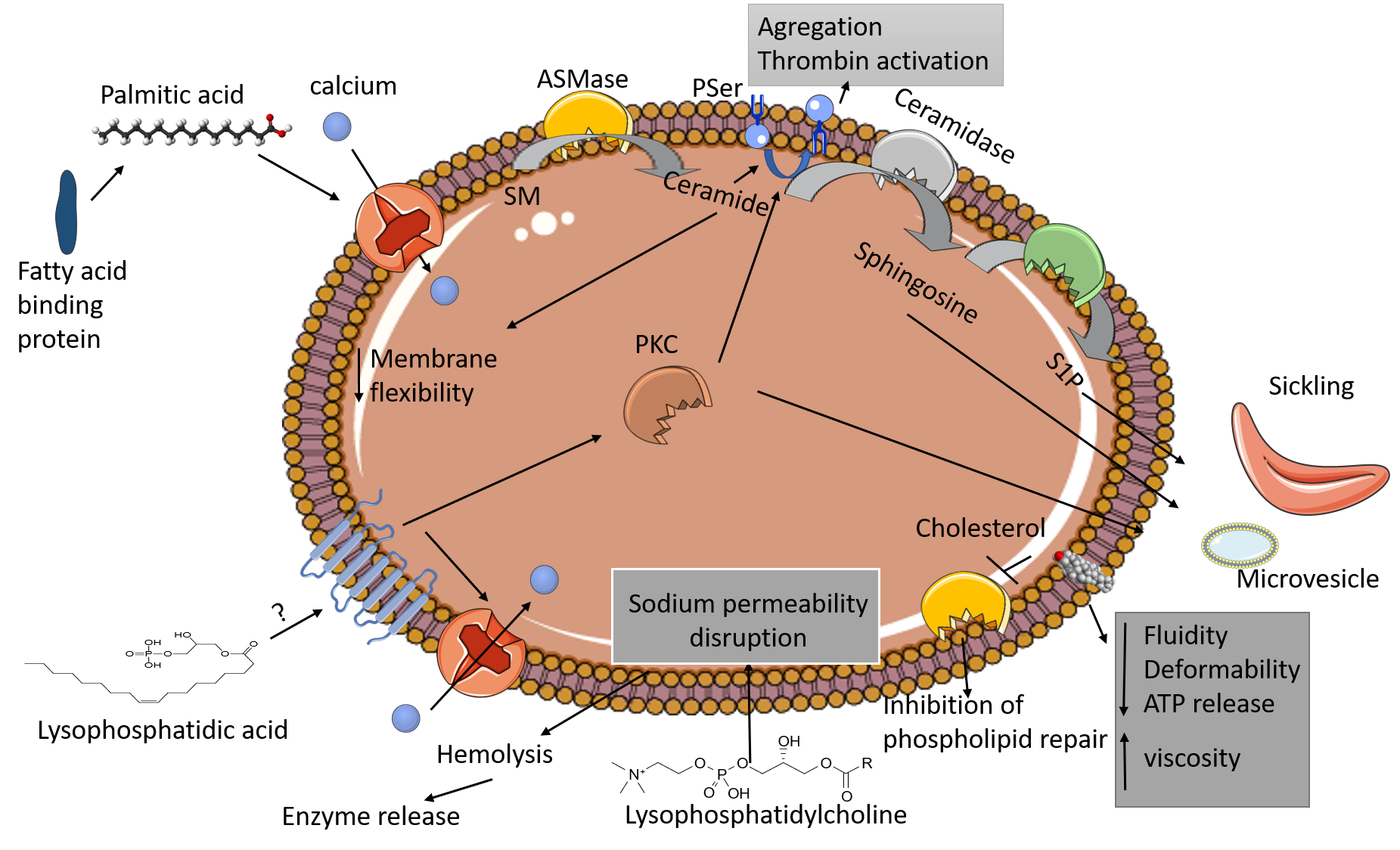
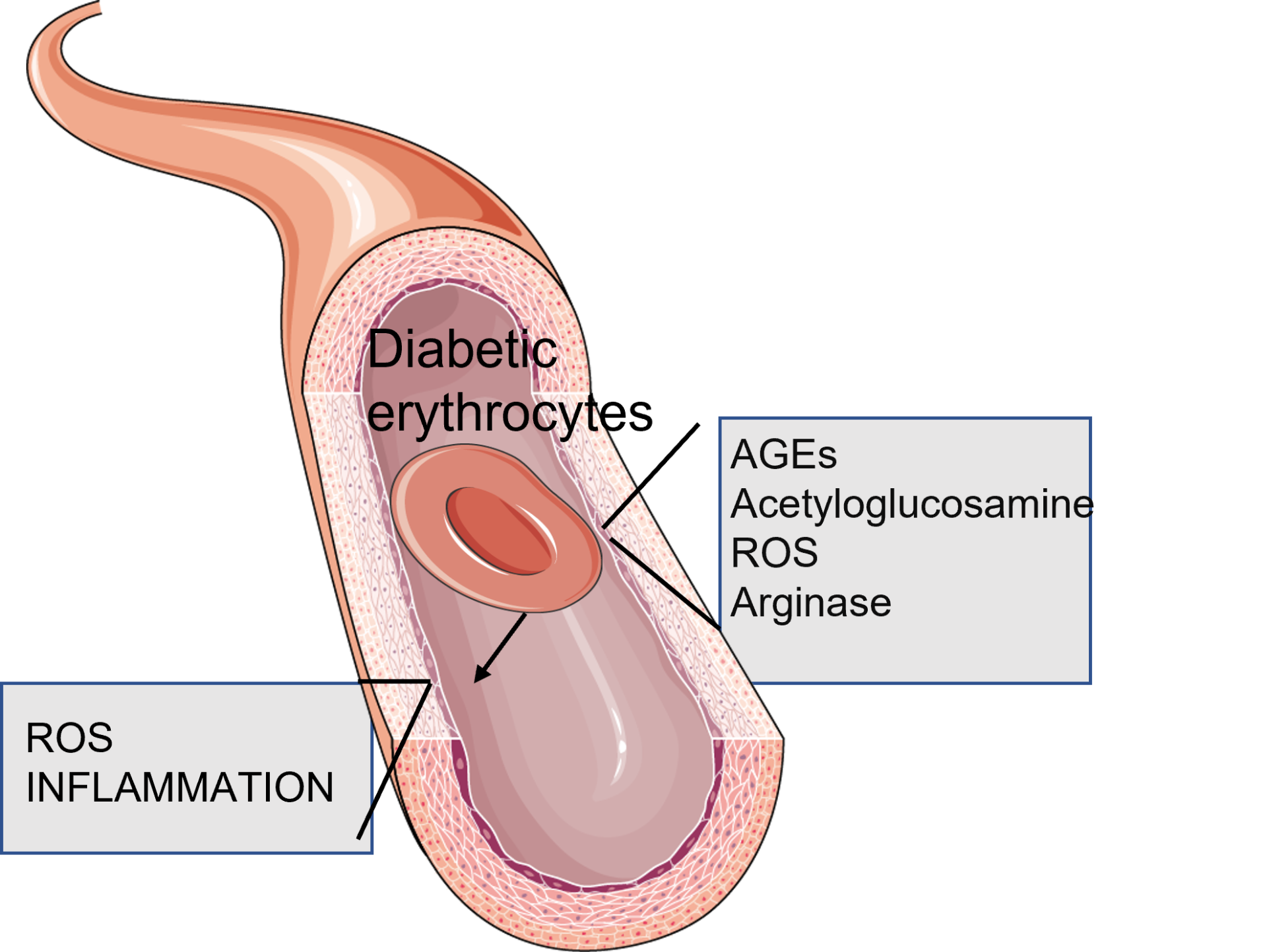